Disusun Ulang Oleh:
Arip Nurahman
Department of Physics
Faculty of Sciences and Mathematics, Indonesia University of Education
and
Follower Open Course Ware at Massachusetts Institute of Technology
Cambridge, USA
Department of Physics
http://web.mit.edu/physics/
http://ocw.mit.edu/OcwWeb/Physics/index.htm
&
Aeronautics and Astronautics Engineering
http://web.mit.edu/aeroastro/www/
http://ocw.mit.edu/OcwWeb/Aeronautics-and-Astronautics/index.htm
Potential applications
Single-molecule gas detection
Theoretically graphene makes an excellent sensor due to its 2D structure. The fact that its entire volume is exposed to its surrounding makes it very efficient to detect adsorbed molecules. However, similar to carbon nanotubes, graphene has no dangling bonds on its surface. Gaseous molecules cannot be readily adsorbed onto graphene surface. So intrinsically graphene is insensitive. The sensitivity of graphene chemical gas sensors can be dramatically enhanced by functionalizing graphene, for example, coating with a thin layer of certain polymers. The thin polymer layer acts like a concentrator that absorbs gaseous molecules. The molecule absorption introduces a local change in electrical resistance of graphene sensors. While this effect occurs in other materials, graphene is superior due to its high electrical conductivity (even when few carriers are present) and low noise which makes this change in resistance detectable.
Graphene nanoribbons
Graphene nanoribbons (GNRs) are essentially single layers of graphene that are cut in a particular pattern to give it certain electrical properties. Depending on how the un-bonded edges are configured, they can either be in a zigzag or armchair configuration. Calculations based on tight binding predict that zigzag GNRs are always metallic while armchairs can be either metallic or semiconducting, depending on their width.
However, recent density functional theory calculations show that armchair nanoribbons are semiconducting with an energy gap scaling with the inverse of the GNR width. Indeed, experimental results show that the energy gaps do increase with decreasing GNR width. However, as of February 2008, no experimental results have measured the energy gap of a GNR and identified the exact edge structure. Zigzag nanoribbons are also semiconducting and present spin-polarized edges.
Their 2D structure, high electrical and thermal conductivity, and low noise also make GNRs a possible alternative to copper for integrated circuit interconnects. Some research is also being done to create quantum dots by changing the width of GNRs at select points along the ribbon, creating quantum confinement.
Graphene transistors
Due to its high electronic quality, graphene has also attracted the interest of technologists who see it as a way of constructing ballistic transistors. Graphene exhibits a pronounced response to perpendicular external electric fields, allowing one to build FETs (field-effect transistors). In their 2004 paper, the Manchester group demonstrated FETs with a "rather modest" on-off ratio of ~30 at room temperature. In 2006, Georgia Tech researchers, led by Walter de Heer, announced that they had successfully built an all-graphene planar FET with side gates. Their devices showed changes of 2% at cryogenic temperatures.
The first top-gated FET (on-off ratio of <2) was demonstrated by researchers of AMICA and RWTH Aachen University in 2007. Graphene nanoribbons may prove generally capable of replacing silicon as a semiconductor in modern technology.
Facing the fact that current graphene transistors show a very poor on-off ratio, researchers are trying to find ways for improvement. In 2008, researchers of AMICA and University of Manchester demonstrated a new switching effect in graphene field-effect devices. This switching effect is based on a reversible chemical modification of the graphene layer and gives an on-off ratio of greater than six orders of magnitude. These reversible switches could potentially be applied to nonvolatile memories.
In 2009, researchers at the Politecnico di Milano demonstrated four different types of logic gates, each composed of a single graphene transistor. In the same year, the Massachusetts Institute of Technology researchers built an experimental graphene chip known as a frequency multiplier. It is capable of taking an incoming electrical signal of a certain frequency and producing an output signal that is a multiple of that frequency. Although these graphene chips open up a range of new applications, their practical use is limited by a very small voltage gain (typically, the amplitude of the output signal is about 40 times less than that of the input signal). Moreover, none of these circuits was demonstrated to operate at frequencies higher than 25 kHz.
In February 2010, researchers at IBM reported that they have been able to create graphene transistors with an on and off rate of 100 gigahertz, far exceeding the rates of previous attempts, and exceeding the speed of silicon transistors with an equal gate length. The 240 nm graphene transistors made at IBM were made using extant silicon-manufacturing equipment, meaning that for the first time graphene transistors are a conceivable—though still fanciful—replacement for silicon.
Graphene optical modulators
When the Fermi level of graphene is tuned, the optical absorption of graphene can be changed. In 2011, researchers at UC Berkeley reported the first graphene-based optical modulator. Operating at 1.2 GHz without any temperature controller, this modulator has a broad bandwidth (from 1.3 to 1.6 μm) and small footprint (~25 μm2).
Integrated circuits
Graphene has the ideal properties to be an excellent component of integrated circuits. Graphene has a high carrier mobility, as well as low noise, allowing it to be used as the channel in a FET. The issue is that single sheets of graphene are hard to produce, and even harder to make on top of an appropriate substrate. Researchers are looking into methods of transferring single graphene sheets from their source of origin (mechanical exfoliation on SiO2 / Si or thermal graphitization of a SiC surface) onto a target substrate of interest.[141]
In 2008, the smallest transistor so far, one atom thick, 10 atoms wide was made of graphene.[142] IBM announced in December 2008 that they fabricated and characterized graphene transistors operating at GHz frequencies.[143] In May 2009, an n-type transistor was announced meaning that both n and p-type transistors have now been created with graphene.[144] A functional graphene integrated circuit was also demonstrated – a complementary inverter consisting of one p- and one n-type graphene transistor.[145]
However, this inverter also suffered from a very low voltage gain.
According to a January 2010 report,[146] graphene was epitaxially grown on SiC in a quantity and with quality suitable for mass production of integrated circuits. At high temperatures, the Quantum Hall effect could be measured in these samples. See also the 2010 work by IBM in the transistor section above in which they made 'processors' of fast transistors on 2-inch (51 mm) graphene sheets.[137][138]
In June 2011, IBM researchers announced[147] that they had succeeded in creating the first graphene-based integrated circuit, a broadband radio mixer. The circuit handled frequencies up to 10 GHz, and its performance was unaffected by temperatures up to 127 degrees Celsius.
Electrochromic devices
Graphene oxide can be reversibly reduced and oxidized using electrical stimulus. Controlled reduction and oxidation in two-terminal devices containing multilayer graphene oxide films are shown to result in switching between partially reduced graphene oxide and graphene, a process which modifies the electronic and optical properties. Oxidation and reduction are also shown to be related to resistive switching. [148] [149]
Transparent conducting electrodes
Graphene's high electrical conductivity and high optical transparency make it a candidate for transparent conducting electrodes, required for such applications as touchscreens, liquid crystal displays, organic photovoltaic cells, and organic light-emitting diodes. In particular, graphene's mechanical strength and flexibility are advantageous compared to indium tin oxide, which is brittle, and graphene films may be deposited from solution over large areas.[150][151]
Large-area, continuous, transparent, and highly conducting few-layered graphene films were produced by chemical vapor deposition and used as anodes for application in photovoltaic devices. A power conversion efficiency (PCE) up to 1.71% was demonstrated, which is 55.2% of the PCE of a control device based on indium-tin-oxide.[152]
Organic light-emitting diodes (OLEDs) with graphene anodes have also been demonstrated.[153] The electronic and optical performance of devices based on graphene are shown to be similar to devices made with indium-tin-oxide.
An all carbon-based device called a light-emitting electrochemical cell (LEC) was demonstrated with chemically derived graphene as the cathode and the conductive polymer PEDOT as the anode by Matyba et al.[154] Unlike its predecessors, this device contains no metal, but only carbon-based electrodes. The use of graphene as the anode in LECs was also verified in the same publication.
Reference material for characterizing electroconductive and transparent materials
One layer of graphene absorbs 2.3 % of white light.[155] This property was used to define the Conductivity of Transparency that combines the sheet resistance and the transparency. This parameter was used to compare different materials without the use of two independent parameters.[156]
Thermal management materials-Thermal interfacial materials
In 2011, researchers in Georgia Institute of Technology reported that a three-dimensional vertically aligned functionalized multilayer graphene architecture can be an approach for graphene-based thermal interfacial materials (TIMs) with superior equivalent thermal conductivity and ultra-low interfacial thermal resistance between graphene and metal.[157]
Solar cells
The USC Viterbi School of Engineering lab reported the large scale production of highly transparent graphene films by chemical vapor deposition in 2008. In this process, researchers create ultra-thin graphene sheets by first depositing carbon atoms in the form of graphene films on a nickel plate from methane gas. Then they lay down a protective layer of thermoplastic over the graphene layer and dissolve the nickel underneath in an acid bath. In the final step they attach the plastic-protected graphene to a very flexible polymer sheet, which can then be incorporated into an OPV cell (graphene photovoltaics). Graphene/polymer sheets have been produced that range in size up to 150 square centimeters and can be used to create dense arrays of flexible OPV cells. It may eventually be possible to run printing presses laying extensive areas covered with inexpensive solar cells, much like newspaper presses print newspapers (roll-to-roll)[158][159]
Ultracapacitors
Due to the extremely high surface area to mass ratio of graphene, one potential application is in the conductive plates of ultracapacitors. It is believed that graphene could be used to produce ultracapacitors with a greater energy storage density than is currently available.[160]
Graphene biodevices
Graphene's modifiable chemistry, large surface area, atomic thickness and molecularly-gatable structure make antibody-functionalized graphene sheets excellent candidates for mammalian and microbial detection and diagnosis devices.[161]
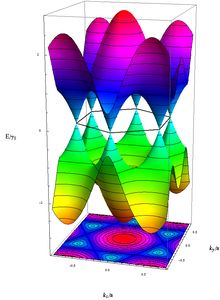
Anti-bacterial
The Chinese Academy of Sciences has found that sheets of graphene oxide are highly effective at killing bacteria such as Escherichia coli. This means graphene could be useful in applications such as hygiene products or packaging that will help keep food fresh for longer periods of time.[163]
External links
- Most of graphene papers published by Andre Geim's group are downloadable here
- Is Graphene the New Silicon? National Science Foundation, March 27, 2008
- "Band structure of graphene" (PDF). http://www.nanohub.org/resource_files/2005/12/00723/2004.10.20-l21-ece453.pdf. Retrieved 2009-08-15.
- Graphene portal with daily news and resources
- Antonio H. Castro Neto (12 May 2009). "Pauling’s dreams for graphene". http://physics.aps.org/articles/v2/30.
- N M R Peres and R M Ribeiro (2009). "Focus on Graphene". New Journal of Physics 11 (9): 095002. Bibcode 2009NJPh...11i5002P. doi:10.1088/1367-2630/11/9/095002.
- Online short course: Colloquium on Graphene Physics and Devices
- List of Authority Articles on Graphene Theme
- Short video explanation 'What is Graphene?'
- Short video 'Graphene and the Carbon Revolution'
- 'Polish team claims leap for wonder material graphene'
- 'Scientists Find Simple Way to Produce Graphene (THE CLAIM of DISCOVERY BY THE AUTHORS IS ERRONEOUS. THEY HAVE BEEN INFORMED OF THIS AS OF AUGUST 8 2011
- 'Graphene technology moves closer'
- 'What Graphene offers for future Electronic Devices by Linda Rae'
- 'Nobel Prize Winners Use Keithley'
- 'Graphene Shows Promise: Reserachers are Looking for New Materials that can take over where Silicon will Leave Off'
- 'How To Avoid Self-Heating Effects on Nanoscale Devices'
- 'Defects Improve Graphene Conductivity'
- 'Rice University lab shows troop how any carbon source can become valuable graphene'
Edited:
November 2011